Introduction
With the intensification of climate change, plants around the world are increasingly exposed to extreme temperatures. Whether it manifests as heatwaves, frosts, or unexpected temperature fluctuations, this phenomenon poses a serious threat to agriculture, food security, and natural ecosystems. Extreme temperature events can lead to a significant reduction in plant growth and reproductive success, resulting in decreased agricultural yield and productivity. To mitigate these effects, it is crucial to understand the physiological, molecular, and genetic mechanisms that plants employ to adapt to extreme temperatures. This article examines these adaptive responses and discusses strategies to enhance plant resilience to rising global temperatures.
Physiological and Morphological Adaptations to Extreme Temperatures
Plants respond to extreme temperatures through a range of physiological mechanisms aimed at minimizing damage while maintaining metabolic functions. One of the first lines of defense against heat stress is the regulation of stomata. When plants are exposed to high temperatures, the stomata close to reduce water loss through transpiration. However, this action also limits CO₂ uptake, disrupting photosynthesis and ultimately inhibiting growth.
Photosynthesis under heat stress
Photosynthetic processes are particularly sensitive to extreme temperatures. Essential enzymes for photosynthesis, such as Rubisco, become less efficient under heat stress conditions. For example, research has shown that temperatures above 35°C in maize significantly reduce photosynthetic efficiency, leading to decreased energy production and compromising the plant’s growth potential. Moreover, stomatal closure under heat stress further restricts CO₂ intake, exacerbating the disruption of photosynthesis. This results in a cascade of negative effects, including reduced biomass and crop yields.
Osmotic regulation and water relations
During drought conditions, plants also exhibit morphological changes, such as altering the root-to-shoot ratio. This change allows plants to prioritize water uptake from deeper soil layers. Some species accumulate osmolytes, such as proline, glycine betaine, and sugars, to maintain cellular turgor pressure, which is crucial for plant survival during heat stress and water shortages. This osmotic regulation helps plants avoid dehydration and maintain cellular functions even in water-limited conditions.
Leaf scorching and premature senescence
Another physiological response to heat stress is leaf scorching and premature senescence, commonly observed during extreme temperatures. When plants reach their tolerance limits, high temperatures can lead to the degradation of cell membranes, resulting in visible symptoms such as leaf scorching. In maize, heat stress during critical growth stages (e.g., pollination) can accelerate senescence, and under water deficit conditions, yield reductions of up to 80-90% can occur.
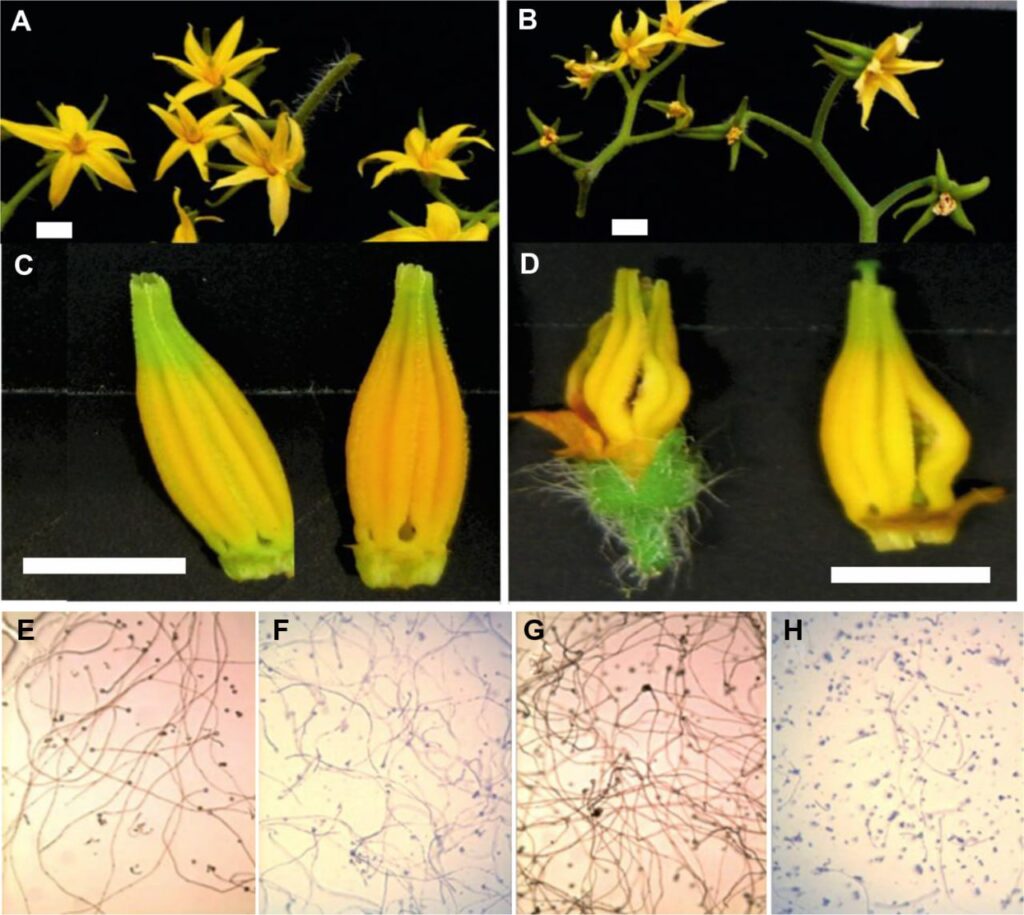
Molecular and Genetic Responses to Extreme Temperatures
At the molecular level, plants respond to temperature stress by activating a set of genes that play a role in stress tolerance. These mechanisms help plants cope with damage and maintain homeostasis.
Heat shock proteins and antioxidants
One of the key defense mechanisms plants employ in response to heat stress is the production of heat shock proteins (HSPs). These proteins act as molecular chaperones, stabilizing cellular proteins and preventing their denaturation under extreme heat, thus helping to maintain cellular structures and protect metabolic pathways from heat-induced damage. Similarly, plants produce antioxidants to reduce the harmful effects of reactive oxygen species (ROS) that accumulate under heat and drought stress. Antioxidants such as superoxide dismutase and catalase eliminate reactive oxygen species and prevent oxidative damage to cellular components like lipids, proteins, and DNA.
Transcriptional regulation and epigenetic changes
Plants use transcription factors to regulate gene expression in response to stress. For example, DREB proteins (dehydration-responsive element-binding) play a crucial role in regulating genes associated with drought and heat responses. In addition, epigenetic changes such as DNA methylation also contribute to stress adaptation by regulating gene expression. This flexible gene regulation system allows plants to quickly respond to environmental stressors, including extreme temperatures.
Genetic diversity in heat tolerance
Genetic diversity within plant species plays a significant role in determining their ability to withstand extreme temperatures. For instance, studies on maize and rice have shown that heat-resistant genotypes can maintain pollen viability and reproductive success even in high temperatures. Pollen development is highly sensitive to extreme temperatures, and in temperatures above 35°C, maize and rice experience reduced pollen survival, leading to a decline in seed formation and crop yield. However, some genetic variants are more resilient and maintain productivity despite heat stress.
Breeding programs and genetic engineering focus on exploiting this natural genetic diversity to enhance heat and drought tolerance in crops. Marker-assisted selection and genome mapping have been used to identify heat tolerance traits, especially in crops like maize and rice, whose reproductive stages are highly sensitive to extreme temperatures.
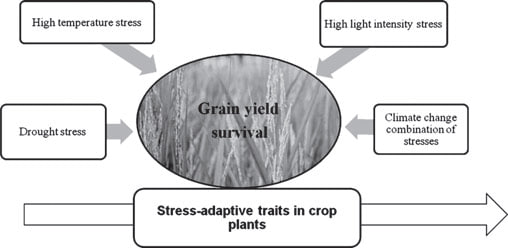
Impacts of Climate Change on Agricultural Productivity and Adaptation Strategies
The impacts of climate change, including rising global temperatures, an increasing number of heatwaves, and shifting rainfall patterns, are becoming increasingly evident, and these factors are expected to have severe consequences for crop production. The Intergovernmental Panel on Climate Change (IPCC) predicts that by 2050, global temperatures will rise by 2 to 3°C, which will result in significant reductions in agricultural productivity, especially in regions reliant on rain-fed agriculture.
Reduced yields
Extreme temperature events, such as heatwaves, accelerate the phenological development of plants, shortening the life cycle of many crops. Faster development usually leads to smaller plants, reduced biomass, and lower reproductive success. Studies show that if current temperature rise trends continue, maize and rice yields may decline by 10-40% in some areas. For example, maize yields are particularly affected by high nighttime temperatures, which accelerate aging and shorten grain-filling periods, resulting in an overall decrease in productivity.
Impact of water stress
The interaction between heat stress and water availability further exacerbates yield reductions. Heat stress increases transpiration rates, and under drought conditions, plants quickly experience water stress, limiting their ability to maintain turgor pressure and intensifying physiological damage. The combined effects of heat and drought stress are often more severe than either factor alone. For instance, maize exposed to high temperatures and reduced water has shown up to a two-thirds reduction in biomass compared to normal conditions.
Adaptation strategies
To cope with the effects of climate change and extreme temperatures, various adaptation strategies are being developed. One promising approach is the development of heat-resistant crop varieties through conventional breeding and genetic engineering. Transgenic plants that enhance the production of heat shock proteins and antioxidant enzymes have shown better survival and productivity under heat stress conditions. Additionally, breeding efforts are focused on traits such as delayed flowering during heat events or strengthening root systems to improve water uptake efficiency.
Other agricultural strategies include adjusting planting times to avoid peak heat periods, using irrigation systems to reduce heat and water stress, and adopting conservation tillage methods to preserve soil moisture. Researchers are also exploring the use of wild relatives of crops, which often possess greater heat and drought tolerance traits, as genetic resources for breeding programs.
Conclusion
Extreme temperatures caused by global climate change present a significant challenge to plant growth and agricultural productivity. While plants have developed a range of physiological, molecular, and genetic adaptations to cope with heat and drought stress, the severity and frequency of these events exceed their natural resilience. Understanding the fundamental mechanisms of temperature tolerance is crucial for developing heat-resistant crop varieties that can withstand the stresses induced by climate change.
By leveraging advances in genetic research, plant breeding, and agricultural practices, it is possible to enhance crop resilience to extreme temperatures and ensure food security for future generations. However, to effectively address the challenges posed by climate change, a multifaceted approach that combines innovative breeding techniques, genetic engineering, and sustainable agricultural practices is essential.
Reference
1. Drought stress adaptation: metabolic adjustment and regulation of gene expression
2. Plant tolerance to high temperature in a changing environment: scientific fundamentals and production of heat stress-tolerant crops. Craita E.Bita and Tom Gerats
3. Temperature extremes: Effect on plant growth and development Jerry L.Hatfield, JohnH.Prueger