Introduction
Root system architecture is a fundamental aspect of plant biology that determines a plant’s ability to absorb water and nutrients from the soil, adapt to environmental stresses, and optimize growth and production. Root system architecture refers to the spatial configuration of roots in the soil, including branching patterns, lengths, diameters, and their density. The interactions between root system architecture and the surrounding soil matrix are crucial for understanding how plants respond to drought stress and nutrient limitations. Recent research, utilizing advanced imaging and modeling techniques, has significantly enhanced our knowledge of the dynamic and variable nature of root system architecture. This article combines the findings of four key studies that investigate the role of root architecture in water absorption, the impact of soil compaction, and the integration of innovative technologies in root system analysis.
Arbuscular Mycorrhizal Fungi and Increased Drought Tolerance
The symbiotic relationship between plants and arbuscular mycorrhizal fungi plays a key role in enhancing plant resilience to drought stress by improving root system architecture. In a study conducted on barley, arbuscular mycorrhizal fungi significantly increased drought resistance by expanding the effective root surface area through extensive hyphal networks. These hyphae, acting as extensions of the root system, penetrate soil micropores that would otherwise be inaccessible to the roots, thus increasing water absorption efficiency.
Quantitative measurements showed that barley plants inoculated with arbuscular mycorrhizal fungi, compared to non-mycorrhizal barley plants, exhibited less reduction in leaf turgor rates, higher stomatal conductance, and more stable net photosynthetic activity under drought conditions. The presence of arbuscular mycorrhizal fungi also helped maintain higher turgor pressure, which is critical for cellular function and growth under water-limiting conditions. These findings highlight the importance of the symbiotic relationship in modifying root architecture to optimize water uptake during periods of water scarcity and suggest a potential strategy for developing drought-resistant crop varieties.
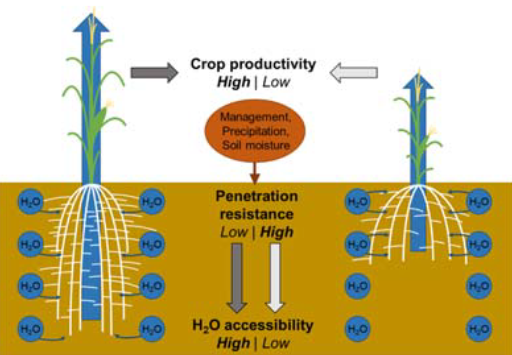
Soil Penetration Resistance as a Limiting Factor for Root Architecture
A study on maize root systems examined the complex feedback mechanisms between soil penetration resistance and root architecture, which significantly impact water absorption and plant growth. As soil compaction increases, a physical barrier is created, limiting root elongation and forcing roots to grow laterally in the upper soil layers. This phenomenon results in shallow root architecture, which hinders the plant’s ability to access deeper water resources during dry periods.
Measurements under various soil conditions showed that maize grown in compacted soil experienced a significant reduction in root depth, with most of the root biomass concentrated in the upper soil layers. This restricted root distribution leads to greater dependence on surface soil moisture, which rapidly diminishes during drought, exacerbating soil dryness and further increasing soil penetration resistance. The study identified this cycle as a “vicious circle,” where increased soil resistance leads to reduced root penetration and limitations in water uptake, ultimately resulting in lower crop yields. These findings suggest that managing soil compaction and increasing soil porosity are essential strategies for promoting deeper root growth and improving water access and drought resilience in crops.
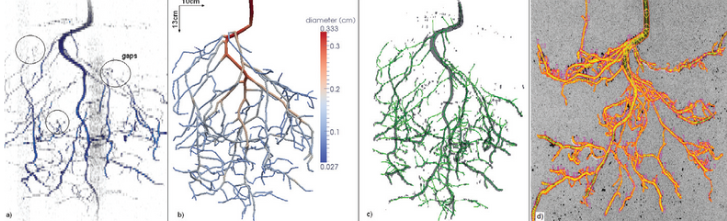
High-Resolution Imaging and Computational Modeling of Root Systems
Advances in non-invasive imaging techniques, such as Magnetic Resonance Imaging (MRI), have revolutionized the study of root system architecture, providing detailed insights into root morphology and function. The use of MRI in a study of lupin roots enabled the three-dimensional reconstruction of the root system, revealing a complex network of root segments and their connections. Automated and semi-manual reconstructions were compared, where the automated methods effectively captured the complexity of the root system with high accuracy and efficiency.
This study employed the Doussan model to simulate water uptake, utilizing data from both semi-manual and automated root system reconstructions. The results indicated that differences in the connectivity of root segments directly influenced the distribution of water flow and xylem pressures within the root network. Automated reconstruction showed a slight decrease in water flow rates compared to the semi-manual method, likely due to variations in segment connectivity. These observations underscore the critical role of accurate root system modeling in predicting water uptake dynamics, especially under varying soil moisture conditions.
The MRI-based automated method allowed for continuous observation of root growth and adaptation in response to environmental stressors. This study highlighted the need to refine computational models to better represent the biological characteristics of root systems, enhancing the ability to predict plant responses to drought and nutrient limitations. The precision of MRI in distinguishing fine root structures and its integration with computational models, such as the Doussan model, can pave the way for more robust simulations of plant-water relationships.
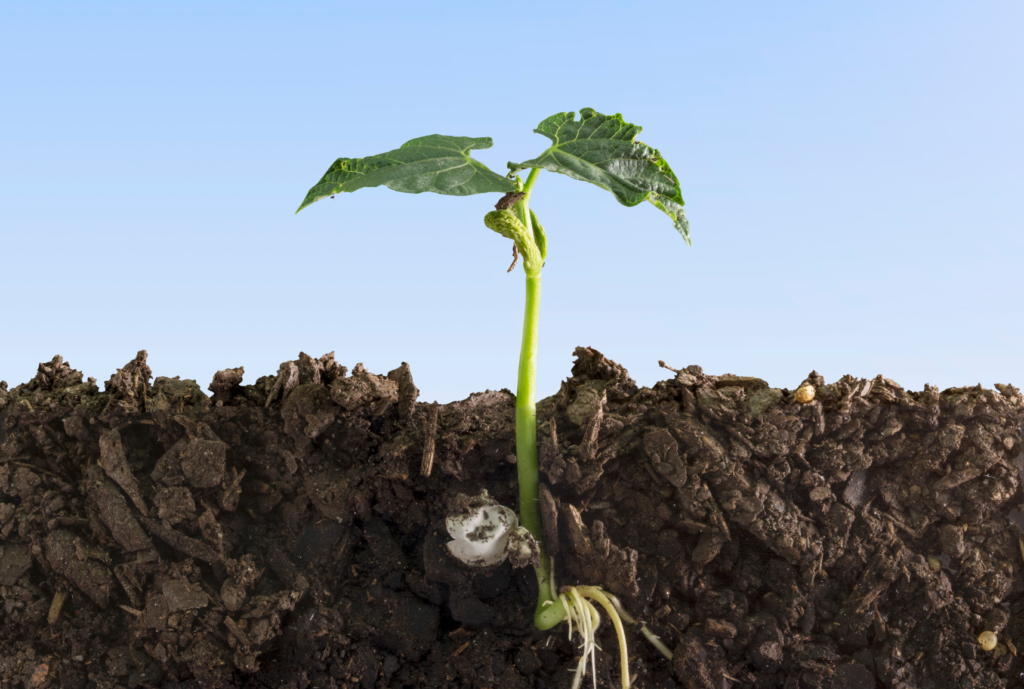
Conclusion
The compilation of research findings on root system architecture highlights its central role in mediating water absorption and plant resilience to environmental stresses. Arbuscular mycorrhizal fungi significantly enhance the study of root system architecture by expanding the root surface and improving water and nutrient uptake, making them a critical component in drought resistance strategies. The feedback loop between soil penetration resistance and root growth in maize exemplifies the challenges of optimizing root architecture under compacted soil conditions and underscores the importance of soil management in crop production.
Additionally, the integration of high-resolution MRI with advanced computational modeling represents a major leap in our ability to visualize and understand the dynamics of root system architecture. These technologies provide unparalleled insights into root behavior and enable more accurate predictions of plant performance under varying soil and climatic conditions. Future research should focus on improving these imaging and modeling techniques to include more precise biological data, ultimately leading to better agricultural practices and the development of crop varieties with greater drought tolerance and nutrient efficiency.
Understanding and optimizing root architecture is not only essential for increasing crop yields but also for efficient water use. It plays a vital role in developing sustainable agricultural systems that can withstand the challenges posed by climate change and resource limitations.
References
1. Quantification of Water Uptake by Arbuscular Mycorrhizal Hyphae and its Significance for Leaf Growth,Water Relations, and Gas Exchange of Barley Subjected to Drought Stress M. A. Khalvati, Y. Hu, A. Mozafar, and U. Schmidhalter Chair of Plant Nutrition, Technical University of Munich, Am Hochanger 1, 85350 Freising-Weihenstephan, Germany Received: April 18, 2005; Accepted: September 1, 2005
2. Feedbacks between soil penetration resistance, root architecture and water uptake limit water accessibility and crop growth – A vicious circle Tino Colombi , Lorena Chagas Torres , Achim Walter , Thomas Keller.
3. Characterizing roots and water uptake in a ground cover rice production system Sen Li, Qiang Zuo, Xiaoyu Wang, Wenwen Ma, Xinxin Jin, Jianchu Shi, Alon Ben-Gal
4. In Situ Root System Architecture Extrac on from Magne Resonance Imaging for Water Uptake Modeling